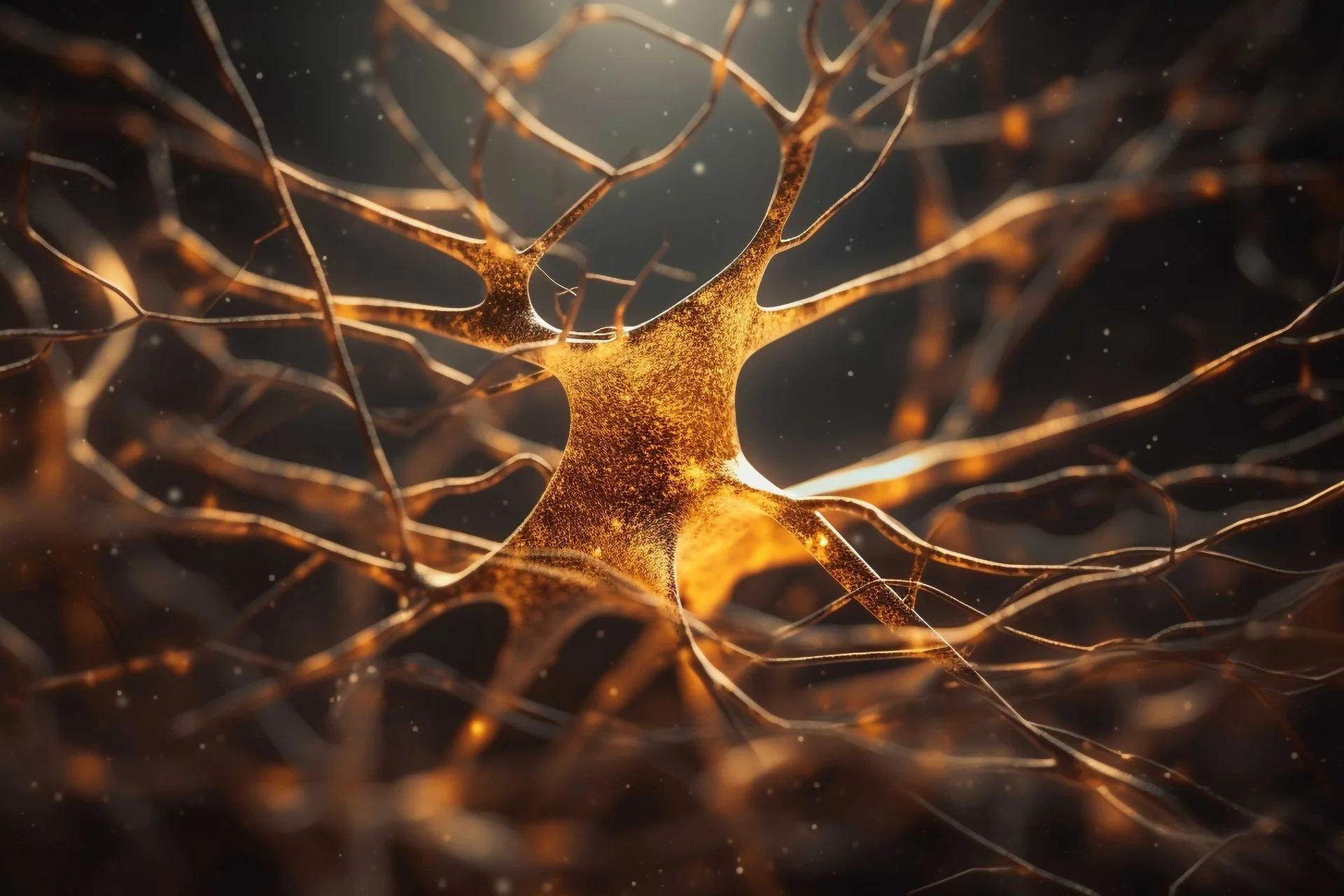
Today, neural tissue engineering, located at the intersection of neural science and engineering fields, has become a new source of hope for the treatment of nervous system diseases and discoveries in neuroscience. In this field, the use of biomaterials continues to be an exciting area of research for the treatment of nervous system diseases and the management of neurological disorders. Biomaterials are widely used in neural tissue engineering because they have biocompatible and bioactive properties. Recent research has made significant progress in this field and has enabled the development of more effective treatment and rehabilitation methods in the future.
Biomaterials are materials that are biocompatible when used inside the body and often interact with biological tissues. These materials can be used in a wide range of medical applications thanks to their biocompatibility and biodegradability. Biomaterials can include a variety of materials, such as biopolymers, hydrogels, bioceramics, biometals, bioelectronic devices, and bioprints. In neural tissue engineering, biomaterials that promote the growth of nerve cells, repair nerve damage, and are compatible with nerve tissue are of great importance. Because biomaterials are compatible and compatible with the human body, they tend to cause minimal immunological reactions when interacting with neural tissues. This feature is critical for acceptance and non-rejection by the body. Biomaterials can promote the growth, regeneration, and repair of neural cells, tissues, and organs. These materials can help restore nervous system functions by allowing neural cells to grow in a suitable environment and integrate into damaged tissue. Biomaterials can be used in various applications in neural tissue engineering. The structures and properties of biomaterials can often be customized. This allows researchers and engineers to customize and optimize materials to suit a specific application or treatment purpose. Biomaterials used in neural tissue engineering are rigorously examined and tested for safety and biocompatibility. It is important that these materials are compatible with the human body and do not cause harmful side effects.
Biomaterial-based nerve repairs
Conditions such as nerve damage, stroke, and traumatic brain injuries are associated with damage to nerve cells. Traditional nerve repair techniques may limit functional recovery after nerve damage. Biomaterials play a critical role in the treatment of such damage. For example, biomaterials such as hydrogels can be used to repair nerve damage. The hydrogel provides an environment that promotes the growth of nerve cells and supports the damaged area. Recent studies show that biomaterial-based approaches offer promising results in this regard. For example, a study conducted at Harvard University showed that a nanofiber-based biomaterial used to repair nerve damage promotes nerve cell regrowth and accelerates nerve repair.
Development of nerve interfaces and prostheses
Artificial nerve interfaces and prostheses have significant potential to restore functions lost due to nerve damage. These devices provide natural nerve signal transmission by enabling the interaction of biomaterials with nerve cells. For example, biomaterial-coated electrodes could help transmit neural signals more effectively in prosthetic arms or legs. Recent research in this field has focused on improving the interaction of biomaterials with nerve cells. For example, a joint study at MIT and Stanford Universities showed that biomaterial-coated electrodes help transmit neural signals more naturally in prosthetic arms and legs.
Drug delivery and treatment of neural diseases
It is important to target drugs used in the treatment of neural diseases effectively and safely. For this purpose, biomaterial-based drug delivery systems are being developed. Biomaterials can be used to develop systems that target drugs to a specific site and provide controlled release. For example, biomaterials containing nanoparticles can achieve targeted drug delivery to nerve tissue. Recent research shows that nanotechnology-based biomaterials can reduce side effects by enabling targeted delivery of drugs used to treat neural diseases.
Recently, innovative production methods such as 3D bioprinting and electrospinning allow the production of more complex biomaterial structures. These methods may expand applications in neural tissue engineering by making it possible to control and customize the properties of biomaterials more precisely.
3D Bioprinting
3D bioprinting is a production technology that enables the creation of 3D structures by combining biological materials layer by layer. In neural tissue engineering, is aimed at producing customized neural tissues and repairing damaged areas using 3D bioprints. This technique makes it possible to create complex structures containing nerve cells, support tissues, and other components. It shows that 3D bioprints have many application potentials in the field of neural tissue engineering. For example, customized 3D bioprints can be used to produce neural implants. These implants can support damaged nerve tissue and promote its repair. Additionally, artificial neural networks, in which nerve cells form complex connections, are also being developed using 3D bioprints.
Electrospinning method
Electrospinning is a technique that studies the effect of electric fields on the growth and differentiation of neural cells. This method demonstrates that electric fields can affect neural cells' orientation, morphology, and function. The electrospinning method can be used to promote the repair of damaged nerve tissue. In recent years, there has been an increase in the number of studies conducted using the electrospinning method. These studies show that electrical stimulation promotes nerve cell regrowth and restores nerve conduction. Additionally, increasing the effectiveness of biomaterials and neural implants using electrospinning is also being investigated. For example, a study using 3D bioprints and electrospinning developed a customized approach to repair damaged nerve tissue. In this study, 3D neural implants produced by bioprinting were integrated into damaged nerve tissue by providing electrical stimulation using the electrospinning method. The results showed that this approach promoted nerve cell regrowth and restored nerve conduction.
The combination of neural tissue engineering and biomaterials holds great promise for the future treatment of nervous system-related diseases and the management of neurological disorders. However, more research and development work is needed in this area. The development of more effective and safe biomaterials could enable neural tissue engineering to have a wider range of applications, which could improve patients' quality of life.
Neural tissue engineering opens a new era in the treatment of nervous system diseases and management of neurological disorders, with the opportunities offered by biomaterials. Advances in this field may enable the development of more effective and personalized treatment approaches in the future and improve patients' quality of life.
Cesur, S., Ulag, S., & Gunduz, O. Microfluidic systems for neural tissue engineering. In Biomaterials for Neural Tissue Engineering (pp. 125-149). Woodhead Publishing. (2023).
Gunduz, O., Ustundag, C. B., & Sengor, M. (Eds.). Biomaterials for Neural Tissue Engineering. Elsevier. (2023).
Yu, X., Zhang, T., & Li, Y. 3D printing and bioprinting nerve conduits for neural tissue engineering. Polymers, 12(8), 1637. (2020).